Advanced Skills and Innovation
Key Messages
- The higher the university completion rate, the higher the share of knowledge-intensive services industries in GDP for Canada and many of its peer countries.
- Countries with a low proportion of business spending on R&D, like Canada, also score “D” grades on the share of PhD graduates.
- Japan demonstrates that colleges can help boost innovation performance. Japan has a high share of graduates from technology and professional training colleges and ranks high on patents per population and the share of world patents.
What types of skills are needed for innovation?
Countries have long recognized that the education and skills of the labour force are the underpinnings of innovation. Innovation is only possible if there are people who can perform research that generates new ways of thinking and new knowledge, who can apply their knowledge and skills, who can adopt new technologies and processes, and who can adapt to change.
Yet there is little hard evidence on the explicit links between specific skills and innovation performance.1 While countries increasingly seek to learn more about the skills needed to improve their innovation performance, they are hampered by a severe lack of quality data to measure skills, as well as data to measure innovation outputs and performance. The OECD notes that more empirical analysis, particularly at the firm level, is needed to better understand the contribution of skills to innovation performance.2
The literature provides little explicit policy guidance. Instead, it identifies a wide range of skills needed for innovation, including basic skills (like reading and writing), technical skills, academic skills, generic skills (like problem solving), creativity, soft skills (like attitudes and behaviours), and management and entrepreneurial skills.3
Firms and industries rely on different skill mixes depending on the stage of innovation, the type of innovation, and the industry structure.4 As a result, according to the OECD, “while there will be differences in the specific skills needed for innovation, in practice, many skills will be relevant across the innovation spectrum.”5 For example, the Conference Board’s Innovation Skills Profile, developed to help employers and employees identify the skills employees need to contribute to their organization’s innovation performance, highlights a range of skills needed, including risk-taking skills, relationship-building skills, and creativity skills.
Why focus on advanced skills?
While a wide range of skills are important for innovation, we have chosen to focus here on examining the link between advanced skills and innovation performance. We know, for example, that making strides in innovation performance requires not only a labour force with advanced skills that enable the development of new technologies, such as information and communications technology (ICT), but also a labour force that can use new technologies effectively. Strong skills also ensure that firms can take full advantage of inward foreign direct investment. The degree to which knowledge-related spillovers from FDI can be exploited and used productively depends on the absorptive capacity of firms. Without an adequately skilled workforce capable of efficiently adapting new technologies and processes, inward FDI will not have as significant an impact as it otherwise might.
To investigate the link between advanced skills and innovation performance, we looked at indicators from the How Canada Performs Education and Skills report card and Innovation report card.
How are advanced skills measured in the How Canada Performs report card?
Education performance is assessed using 15 indicators across three education and skills levels: basic, mainstream, and advanced. People with advanced education and skills have high literacy and job-specific skills as well as advanced thinking skills that enable them to adapt to workplace change, innovate, and create new processes, products, and services.
To assess the relationship between advanced skills and innovation performance, we chose three advanced-skills indicators from our Education report card:
- university completion
- PhD graduates
- science and engineering graduates
How is innovation measured in the How Canada Performs report card?
The Conference Board defines innovation as a process through which economic or social value is extracted from knowledge—through the creation, diffusion, and transformation of knowledge to produce new or significantly improved products or processes that are put to use by society. In other words, innovation is not just about inventing new products and services, it is also about new or improved ways of doing things.
The Conference Board identifies four major types of business innovation: radical change to products and services, radical change to processes, incremental improvement to products and services, and incremental improvement to processes. Innovation includes technological advances, but it also embodies non-technological advances, such as process improvements, new business models, marketing, and branding.
Because of a lack of data on non-technological innovation output, the How Canada Performs innovation report card focuses primarily on technological innovations.6 To assess the relationship between advanced skills and innovation performance, we chose three indicators from our Innovation report card:
- knowledge-intensive services as a share of GDP
- high-technology manufacturing as a share of GDP
- patents per population
Because of the difficulty measuring innovation outputs, we also chose to examine the relationship between advanced skills and:
- an innovation input indicator (R&D expenditures)
- the overall How Canada Performs ranking on innovation
Do countries with high university completion rates also have a larger share of knowledge-intensive services industries and high-technology manufacturing industries?
The data for Canada and its peer countries reveal a positive relationship between university completion and the innovation indicator that measures knowledge-intensive services as a share of GDP. Knowledge-intensive services industries (post and telecommunications, finance and insurance, and business activities) rely on high technology intensively and therefore require a highly skilled labour force to use and exploit technological innovations.7
Canada ranks 10th among 17 peer countries on the knowledge-intensive services indicator and 6th among 17 peer countries on university completion. While university completion rates have increased in Canada, they have also increased in peer countries, explaining Canada’s consistent “B” ranking over the past decade. The U.S., which ranks among the top countries on innovation, also ranks high on university completion: 30 per cent of the U.S. population aged 25 to 64 had a university degree in 2008—this is five percentage points higher than in Canada. If we focus on the younger 25–34 cohort, Canada fares worse, ranking 11th among its peers—not a good sign of Canada’s future performance vis-à-vis its peers.
Use the bubble diagram below to see how the relationship between university completion and the share of knowledge-intensive services has changed over time for Canada and its peer countries. Follow these steps:
- Under “Color,” choose “Country.” That way, each colour will represent a specific country. (When you scroll over each coloured bubble, the country name will pop up.)
- Under “Size,” we recommend you choose “Population” or “Normalized GDP per capita” as the variable to track based on the size of the bubble. That way, you will be able to see at a glance how bigger countries are doing compared with smaller ones, or how richer countries are doing compared with poorer ones.
- Choose “University completion” as the variable to track on the horizontal axis.
- Choose “Knowledge-intensive services” as the variable to track on the vertical axis.
- If you want to see a trail for a particular country, click in the “Trails” box, and then click in the box of the country (or countries) you want to trail. Each country will be represented by a trail of bubbles in its unique colour.
- Finally, click the play button in the bottom left to begin the show.
As seen in the bubble chart, for most of the peer countries, as the share of the population with a university degree has increased over the years, so has the share of knowledge-intensive services in total GDP. Intuitively, this makes sense. The proportion of the population with a university degree has trended upwards for all of the comparator countries. Meanwhile, knowledge-intensive services as a share of GDP has also been growing over the years, with economies around the world becoming increasingly knowledge-based.
One notable exception is Norway, which ranks lowest on the knowledge-intensive services indicator but highest on university completion. Norway’s small share of knowledge-intensive services is due to the fact that the oil and gas industry makes up a disproportionate share of overall GDP in the country.
The situation in Switzerland is the opposite to that of Norway: Switzerland’s knowledge-intensive services industries make up a large proportion of its GDP—Switzerland’s renowned banking industry is the reason behind its strong showing on this indicator. The university attainment of its population, however, is average compared with that of its peers. Switzerland’s education system is somewhat different from that of other OECD countries. A very high proportion of young people complete upper secondary education, but almost 60 per cent of them do so in a dual vocational system, which combines workplace-based, vocational training with one or two days per week of school-based general education. Only 30 per cent of students follow a course that prepares them for entry into university. This may not be an unreasonable choice for Swiss students because, as the OECD aruges, “tertiary attainment [in Switzerland] contributes little to raising the probability of being in employment, reflecting the high employment rates achieved by graduates from vocational upper secondary education.”8 The OECD argues that recent employment rates of university graduates have “evolved more favourably than those of upper secondary vocational graduates. The returns are particularly high for universities of applied sciences and tertiary vocational degrees.”9
The scatter chart below (excluding the two outliers, Norway and Switzerland) shows the positive relationship between average university completion and the average share of knowledge-intensive services in GDP from 2001 to 2005.
We also examined the relationship between university completion and knowledge-intensive services as a share of GDP for all the peer countries over time, between 1998 and 2007 (not taking an average). This analysis—a time-series regression—revealed a strong positive linear relationship (with a correlation coefficient, or R2, of 0.91) between the two variables over time. The strength of the relationship varies among countries.
Focusing on just the U.S. and Canada reveals that both the university completion rate and the knowledge-intensive services share of GDP are lower in Canada.
Sweden ranks relatively high on university completion yet low on knowledge-intensive services industries as a share of total GDP. This is understandable, because its economy is geared more toward high-technology manufacturing than services. In fact, Sweden ranks third among peer countries on high-technology manufacturing as a share of GDP.
Other countries that have a large share of high-technology manufacturing include Ireland and Japan. Neither of these countries has exceptional university completion rates, but they both rank highly on college completion. In Japan, many students pursue vocational training in technology colleges and professional training colleges that better prepare them to join the Japanese labour force. Not surprisingly, Norway, an oil-based economy, ranks close to the bottom of the pack on the share of high-technology manufacturing.
You can use the previous bubble diagram to track the relationship between the countries’ university completion rate or college completion rate and their share of high-technology manufacturing.
Do more PhDs mean more patents and more R&D spending?
Doctorate holders have the highest level of educational attainment and are specifically trained to conduct research, so intuitively it makes sense that countries with a high share of PhD graduates also have a high number of patents per population. A study that looked at the employment of PhD graduates in Ireland found that “R&D active firms employing PhD researchers have rates of patenting 2.5 times greater than similarly active firms which do not employ PhD researchers.”10
On the indicator that measures the number of PhD graduates in a particular year, Canada ranks last among its peers. Canada also ranks poorly—14th out of 17 countries—on the patents by population indicator—a measure of the relative intensity in transforming knowledge into invention.
Use the bubble diagram below to see how the relationship between PhD graduates and patents per population has changed over time for Canada and its peer countries. Follow these steps:
- Under “Color,” choose “Country.” That way, each colour will represent a specific country. (When you scroll over each coloured bubble, the country name will pop up.)
- Under “Size,” we recommend you choose “Population” or “Normalized GDP per capita” as the variable to track based on the size of the bubble. That way, you will be able to see at a glance how bigger countries are doing compared with smaller ones, or how richer countries are doing compared with poorer ones.
- Choose “PhDs” as the variable to track on the horizontal axis.
- Choose “Patents per population” as the variable to track on the vertical axis.
- If you want to see a trail for a particular country, click in the “Trails” box, and then click in the box of the country (or countries) you want to trail. Each country will be represented by a trail of bubbles in its unique colour.
- Finally, click the play button in the bottom left to begin the show.
As seen in the bubble diagram, countries that rank high on patents—such as Switzerland, Sweden, and Germany—are also ones that have a high PhD graduate rate (the number of PhD degrees granted in a particular year per 100,000 population aged 25 to 29).
Japan is the exception, however. Japan ranks second to last on the PhD graduation rate, yet it has the second highest number of patents per population. Japan also ranks second, after the U.S., on the share of world patents indicator.
Why does Japan have such a low PhD graduate rate? Many Japanese choose to enrol in vocational degree programs rather than pursue university degrees. Technology and professional training colleges are popular in Japan because they are considered to be better preparation for joining the workforce and are viewed favourably by employers. Japanese employers tend not to reward graduate study; many, in fact, consider it to be a hindrance since it hampers an employee’s ability to adapt to a given firm’s corporate culture.11 Relative to other countries, graduate students in Japan receive less financial support, so the return on completing a PhD in Japan is lower. According to the OECD, “the economic returns to PhD education [in Japan], on average, appear not to outweigh the opportunity costs of PhD study. As a result, graduate student numbers, though rising, remain modest by international standards.”12
Japan demonstrates that colleges can have a beneficial impact on innovation performance. In the bubble diagram above, if we look at the relationship between college completion (instead of PhD graduates) and patents per population, we see the relationship is clearly a positive one for Japan. In Canada, it has been shown that collaborations between colleges and industry can act as innovation catalysts and accelerators.13
Increased numbers of PhD students and graduates can boost R&D spending in both higher education institutions and businesses through collaborations between universities and industry—provided that research opportunities exist, of course. Canada continues to be a laggard when it comes to business expenditures on R&D (BERD). Interestingly, countries such as Finland, Sweden, and Switzerland that rank high on BERD also rank high on PhD graduates. Countries with a low proportion of BERD—such as Canada, the Netherlands, and Italy—have below-average PhD graduate rates.
Would more science and engineering graduates improve Canada’s innovation performance?
Maybe. Technological innovation activity is typically associated with science, engineering, and related disciplines. Canada is a middle-of-the-pack performer on the indicator that measures the proportion of graduates from science, math, computer science, and engineering programs, scoring a “C” grade and ranking 10th out of 17 peer countries. Other countries that also rank low on both this indicator and overall innovation performance include Italy, Australia, and Norway.
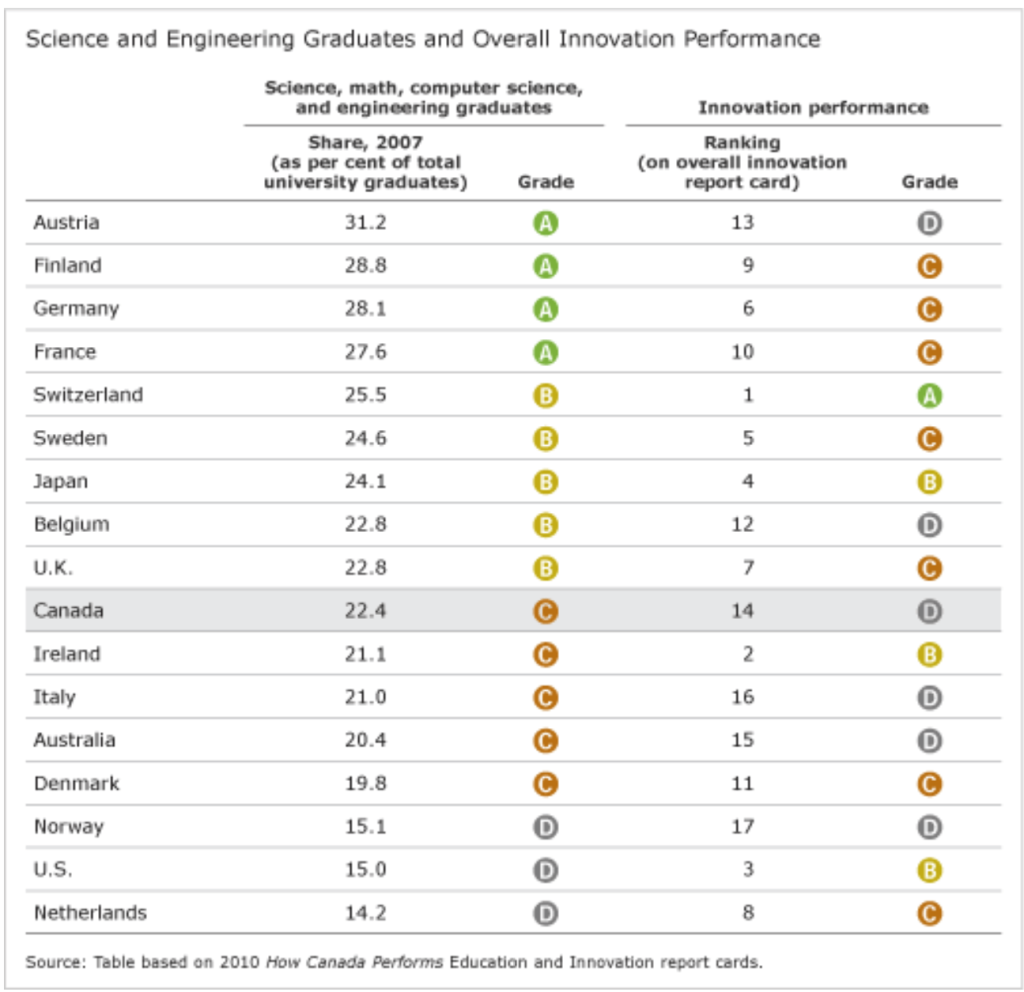
The table shows that two countries, in particular, seem to contradict the notion that a higher proportion of science and engineering graduates leads to better innovation performance: Austria and the United States.
Austria ranks first on the science, math, computer science, and engineering graduates indicator, but scores a “D” grade and ranks 13th out of 17 countries on the overall innovation report card. Why isn’t the large share of science and engineering graduates boosting Austria’s innovation performance? Because this share is calculated in relation to the total number of university graduates in Austria—a number that is very low. So even though the calculated share of science and engineering graduates is high, the actual number of graduates in these disciplines is low. Austria ranks last among its peers on university completion, and has scored a “D” grade on this indicator each year since 1998. France and Belgium also rank high on the share of science and engineering graduates but do poorly on overall university completion.
Interestingly, the U.S., which ranks among the top on the innovation report card, has the second lowest share of science, math, computer science, and engineering graduates among the peer countries. Of course, this does not mean that graduates from these disciplines are not important to the country’s strong innovation performance. True, the U.S. has a low share of science and engineering graduates in relation to its total number of graduates. But university completion rates in the U.S. are higher, and the sheer volume of science and engineering graduates far exceeds the number in Canada. In 2008, there were over 348,000 graduates from science and engineering programs in the U.S.—seven times more than in Canada that year.14 The U.S. also attracts large numbers of science and engineering graduates from other countries—so its relatively low share of graduates in these fields doesn’t mean that people with degrees in these fields are not available in the labour force.
The data on PhD graduates in the fields of science and engineering reveal that Canada has one of the highest proportions of graduates in these fields as a proportion of total PhD graduates. But this by no means implies that Canada is a leader when it comes to the number of advanced degrees awarded in science and engineering, because the total number of PhD graduates in the country is low.
Also, the number of science and engineering PhD graduates may be a misleading indicator of Canada’s innovation potential since a large number of graduates leave Canada to pursue post-doctoral studies or work in the United States. About one-fifth of graduates from PhD programs in 2005 planned to leave Canada upon completion of their degree, with the majority planning to go to the United States.15 Furthermore, the largest share of PhD graduates who leave Canada to go to the U.S. are from the life sciences and from computer, mathematics, and physical sciences. In 2007, a significant proportion of 2005 PhD graduates from Canada living in the U.S. were from the life sciences (17 per cent) and computer, mathematics, and physical sciences (17 per cent).16 Most PhD graduates from Canada move to the U.S. for work-related reasons, with the quality of research facilities or the commitment to research being two top-cited factors attracting graduates.17
What about managerial skills?
Businesses need people with strong managerial skills to drive innovation. Managers develop the business strategies that lead to increased R&D, technology adaptation, and commercialization. Managers foster innovation by adapting to change, making sure their businesses adopt new technologies, ensuring that R&D financing is available, and taking risks. Whether for product or process innovation, managers with the right business acumen are essential to ensuring that it happens.
Are people born managers? Or are managerial skills learned through advanced education? This question is a source of debate. Those with master’s degrees in business administration (MBAs) go through extensive management training, but does that give them the intuitive and risk-taking skills necessary to managing innovative businesses? Or do many of them already have these skills, which is why they are drawn to pursuing an MBA in the first place? Many people without formal management education have gone on to run incredibly successful and innovative companies (Steve Jobs, co-founder and CEO of Apple, and Bill Gates, co-founder of Microsoft, to name a couple).
Data on university graduates from business programs do not reveal whether managers in countries have the specific management skills necessary for innovation. Those with MBAs do not necessarily go on to pursue careers in management. Also, MBA students often study abroad and return to their home country or move elsewhere upon graduation. So, just because a country has a large number of MBA graduates does not mean it has a large number of managers with MBAs. Data on the educational attainment of managers would be useful. Unfortunately, comparable data for Canada and its peer countries are not available. However, data from 2001 and 2005 on educational attainment of managers in Canada and the U.S. show that a greater proportion of U.S. managers are university-educated, and the proportion with business degrees is more than double that in Canada.18
Is a large supply of people with university degrees enough to boost innovation performance?
No. Without the demand for graduates with advanced degrees, an increased supply won’t have much of an impact on innovation performance. If there are no jobs for PhD graduates or science and engineering graduates, then clearly an increased number won’t do much to boost innovation performance. For example, if PhD research opportunities or science and engineering jobs are not available in Canada, then graduates move to where they are available, like the U.S., or end up working in fields not related to their field of study.
Also, skills gained in university are not the only ones that matter. Skills gained through colleges and other vocational training institutes are also important to innovation. Japan, a country renowned for its innovation performance, has a high share of graduates from technology and professional training colleges but a low share of PhD graduates. In Canada, applied research collaborations between colleges and industry are emerging as innovation catalysts and accelerators.19 Furthermore, innovation skills need to be infused in school curricula at all levels of education.20
Finally, while human capital is clearly important to innovation, many factors besides the supply of people with advanced skills influence innovation performance. Having a business environment that fosters innovation is critical. Domestic and foreign competition, a risk-taking culture, and the availability of adequate financing (such as venture capital) all play a role in innovation.
Footnotes
1 OECD, Skills for Innovation and Research (Paris: OECD, 2011), 9.
2 Ibid., 104. To address this lack of firm-level analysis, the Conference Board has created the Centre for Business Innovation (CBI). A five-year initiative, the CBI has a mission to learn why Canada is not a leader in business innovation, to generate evidence and track Canada’s performance, to develop business strategies for firms and capital markets, and to propose public policies that will stimulate business innovation, which is essential to Canada’s competitiveness.
3 OECD, Skills for Innovation and Research (Paris: OECD, 2011), 9, 32–33.
4 Ibid., 10.
5 Ibid.
6 The 2005 Oslo Manual identified two types of innovation that can be considered “not technological”: marketing innovations and organizational innovations. A marketing innovation is the implementation of a new marketing method involving significant changes in product design or packaging, product placement, product promotion, or pricing. An organizational innovation is the implementation of a new organizational method in the firm’s business practices, workplace organization, or external relations. OECD, Oslo Manual: Guidelines for Collecting and Interpreting Innovation Data, 3rd Edition (Paris: OECD, 2005).
7 To identify knowledge-intensive services, the OECD analyzed each industry’s use of technology (based on input-output tables), each industry’s R&D intensity, and the workforce skills of each industry. Based on this analysis, the OECD defines three services industries as “knowledge-intensive”: post and telecommunications, finance and insurance, and business activities.
8 Andrés Fuentes, “Raising Education Outcomes in Switzerland,” Economics Department Working Paper No. 838 (Paris: OECD, 2011), 12.
9 Ibid., 12.
10 Advisory Science Council, The Role of PhDs in the Smart Economy (Dublin: Forfas, December 2009), ix.
11 Howard Newby, Thomas Weko, David Breneman, Thomas Johanneson, and Peter Maassen, OECD Reviews of Tertiary Education—Japan (Paris: OECD, 2009), 64.
12 Ibid., 76.
13 Daniel Munro and Joseph Haimowitz, Innovaton Catalysts and Accelerators: The Impact of Ontario Colleges’ Applied Research (Ottawa: The Conference Board of Canada, November 2010). Available from The Conference Board of Canada.
14 OECD.Stat. (accessed October 10, 2010). Note: Level of education includes Tertiary-Type A and advanced research programs. Fields of education include 400: Science, and 500: Engineering, Manufacturing and Construction, as described by the 1997 International Standard Classification of Education (ISCED-97). Science graduates (ISC40) include students from the field of life sciences (ISC42), physical sciences (ISC44), mathematics and statistics (ISC46), and computing (ISC48); engineering graduates includes the fields of engineering and engineering trade (ISC52), manufacturing and processing (ISC54), and architecture and building (ISC58).
15 Louise Desjardins and Darren King, Expectations and Labour Market Outcomes of Doctoral Graduates from Canadian Universities, (Ottawa: Statistics Canada and Human Resources and Skills Development Canada, January 2011), 21.
16 Ibid., 23.
17 Ibid., 24.
18 Council of Canadian Academies, Innovation and Business Strategy: Why Canada Falls Short (Ottawa: Council of Canadian Academies, June 2009), 61–62.
19 Daniel Munro and Joseph Haimowitz, Innovation Catalysts and Accelerators: The Impact of Ontario Colleges’ Applied Research (Ottawa: The Conference Board of Canada, November 2010). Available from The Conference Board of Canada.
20 Michael Bloom and Douglas Watt, “Solving Canada’s Innovation Conundrum: How Public Education Can Help,” Issue Statement #1 (Ottawa: The Conference Board of Canada, July 2003).